Green hydrogen is gaining a lot of interest as we look for cleaner and more sustainable energy options. With the serious effects of climate change and the urgent need to reduce carbon emissions, green hydrogen is becoming a promising alternative to fossil fuels. But what exactly is green hydrogen, and why does it matter so much?
In this blog post, we’ll explore the world of green hydrogen. We’ll break down what it is, how it’s made, the different types, and the many ways it can be used. We’ll look at the science behind its production, the innovative techniques to create it in an eco-friendly way, and the incredible potential it has to change industries and power our future.
Also Read: Flywheel Energy Storage Explained
What is Hydrogen
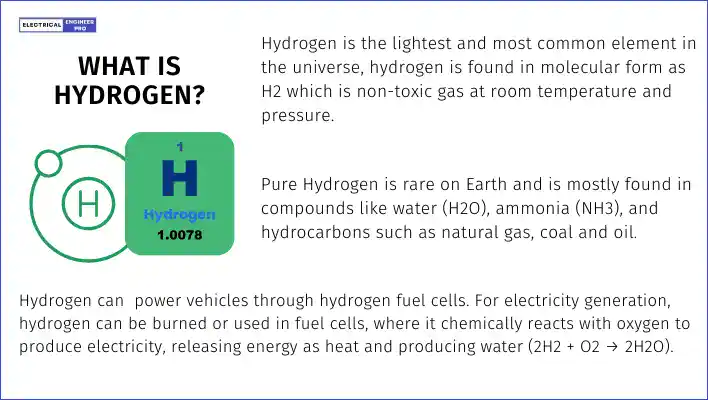
Before we dive into green hydrogen, let’s first understand what hydrogen is and how it fits into the global energy scene.
Hydrogen is the lightest and most common element in the universe. It’s marked by the symbol ‘H’ on the periodic table and has an atomic number of 1. Usually, hydrogen is found in molecular form as H2 which is non-toxic gas at room temperature and pressure. Also, it can be turned into a liquid at very low temperatures.
Due to its high reactivity, pure hydrogen is rare on Earth and is mostly found in compounds like water (H2O), ammonia (NH3), and hydrocarbons such as natural gas, coal and oil.
Unlike natural gas, oil and coal, which are mined from the Earth, hydrogen gas (H2) is not available naturally in large amounts. Instead, hydrogen works as an energy carrier, much like electricity. This means it’s produced from other energy sources like natural gas, coal or renewable sources like solar power.
Once produced, hydrogen has many uses. It can power vehicles through hydrogen fuel cells, which only emit water and heat. For electricity generation, hydrogen can be burned or used in fuel cells, where it chemically reacts with oxygen to produce electricity, releasing energy as heat and producing water (2H2 + O2 → 2H2O).
In the chemical process no carbon is involved, so using hydrogen made from renewable or nuclear energy can help eliminate carbon monoxide and ozone pollution and reduce greenhouse gas emissions. However, burning hydrogen can still create small amounts of nitrogen oxides.
These qualities make hydrogen a clean and versatile energy resource that could significantly reduce pollution and support the use of renewable energy.
Benefits of Hydrogen in Energy Landscape
Hydrogen offers several important benefits as part of the global energy landscape, thanks to its unique features and wide range of applications. Here are the key benefits:
- Clean Burning: Hydrogen burns cleanly, producing only water as a by-product. This makes it a great alternative to fossil fuels, significantly reducing greenhouse gas emissions, especially in transportation.
- Versatility: Hydrogen is incredibly versatile. It can power fuel cell vehicles, which can replace traditional gasoline cars and trucks, lowering emissions in the transport sector. It is also useful for generating electricity, helping integrate renewable energy into the grid and boosting energy storage capabilities.
- High Energy Content: Hydrogen packs a lot of energy per unit of mass, making it an efficient fuel. This high energy density is ideal for energy-intensive applications, like industrial processes and heavy-duty transportation.
- Energy Storage and Grid Stability: Hydrogen can be easily stored and transported, making it a flexible energy carrier. It’s excellent for storing surplus energy from renewable sources like wind and solar, supporting grid stability and providing a reliable backup during periods of low renewable energy generation.
- Support for Renewable Energy: Hydrogen works well with renewable energy sources. Extra electricity from wind or solar power can be used to produce hydrogen through electrolysis. This hydrogen can then be stored and used later, providing a steady energy supply even when wind or solar power is low.
- Energy Security: Hydrogen can improve energy security by diversifying our energy sources and lessening our dependence on fossil fuels. We can produce it locally from resources like natural gas, biomass, and water using renewable electricity.
Good to Know
Hydrogen as Energy Carrier: Hydrogen is called an energy carrier because it needs to be made from other energy sources. Once produced, hydrogen can be stored, transported, and used in many different ways. It can power vehicles with hydrogen fuel cells, make ammonia, create biofuels, and be used in industrial processes like metalworking and welding, among other uses.
Also Read: Energy Storage System | Key Technologies Explained
What is Green Hydrogen
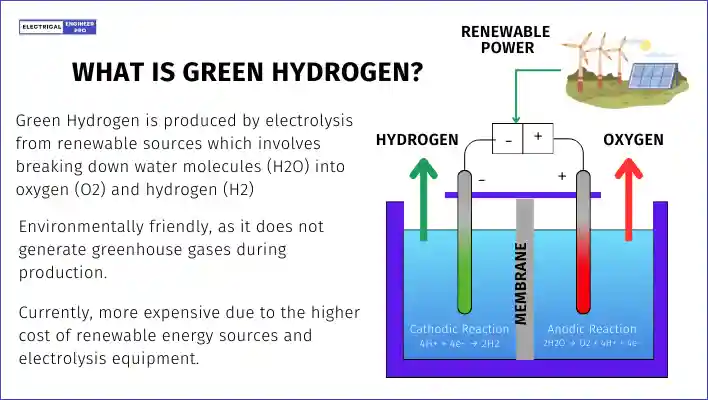
Different countries have different ideas about what counts as renewable energy, how emissions are measured, when hydrogen is considered green, and what methods and materials can be used to make it. As a result, each country or organization has its own interpretation of what constitutes Green Hydrogen.
Let’s look at two examples: one from the Global Hydrogen Organization (GHO) and the other from India’s Ministry of New and Renewable Energy (MNRE) which are widely referred to.
According to GHO, Green Hydrogen is hydrogen produced by splitting water into hydrogen and oxygen using renewable electricity through electrolysis. This process results in very low or zero carbon emissions.
MNRE definition provides a more detailed perspective. It states that Green Hydrogen is produced through electrolysis, where water is split into hydrogen and oxygen using electricity generated from renewable sources like solar, wind, or hydro-power. This method produces a clean and emission-free fuel that has great potential to replace fossil fuels and reduce carbon emissions.
MNRE also mentions another method involving biomass gasification to produce Green Hydrogen, highlighting its versatility and sustainability.
Both definitions agree that Green Hydrogen is produced through electrolysis using renewable electricity. However, MNRE’s definition expands on this by including alternative methods like biomass gasification. This broader view emphasizes the diverse applications of Green Hydrogen beyond just electrolysis.
In summary, while both definitions confirm that Green Hydrogen is made via electrolysis with renewable electricity, MNRE’s definition offers a more comprehensive outlook by covering additional production methods.
From these definitions, it can be concluded that Green hydrogen is a type of hydrogen produced using environmentally responsible methods, such as harnessing wind and solar renewable power, ensuring zero emissions and no harm to the planet.
Also Read: Biomass Energy Explained | Resources, benefits & Tech
Green Hydrogen Production
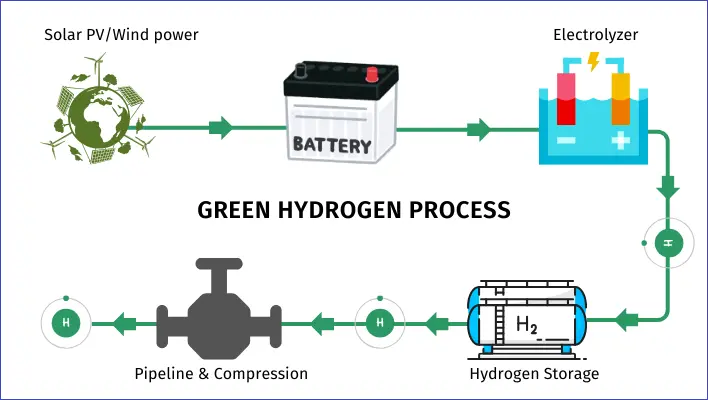
Globally, nearly all (over 99%) of the world’s hydrogen is produced from traditional fossil fuels. Here is the source breakdown,
- Natural Gas (Grey Hydrogen): The predominant source, accounting for about 76% of global hydrogen production. This process is known as Steam Methane Reforming (SMR).
- Coal (Brown Hydrogen): Contributes about 23% of hydrogen production, mainly through coal gasification, which is more common in countries with abundant coal reserves like China.
- Oil (Refinery By-Products): A small percentage of hydrogen is produced as a by-product of oil refining processes.
The main problem with using these sources in power generation is that it leads to greenhouse gas emissions into the atmosphere, accelerating the temperature rise in earth’s surface and triggering climate change.
So Green hydrogen must be produced without generating greenhouse gas (GHG) emissions. There are three major electrolysis technologies at different maturity levels that can produce hydrogen without GHG emissions. They are,
- Alkaline Water Electrolysis (ALK)
- Polymer Electrolyte Membrane (PEM)
- Solid Oxide Electrolyzer Cell (SOEC)
Also Read: Guide to Geothermal Energy | How Earth’s Power is Harnessed?
Alkaline Water Electrolysis (ALK)
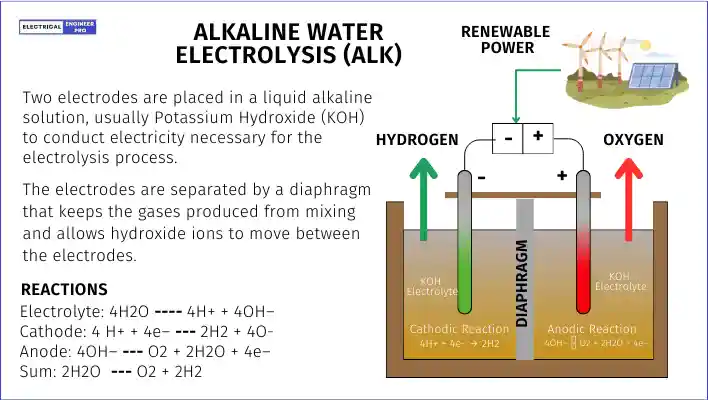
Alkaline water electrolysis (ALK) is the most established method for producing green hydrogen, holding around 70% of this small but growing market. It’s popular because it’s affordable and the equipment lasts a long time.
However, ALK systems need to run continuously as stopping and starting can damage the machinery. This makes it tricky to use with renewable energy sources alone, as they can be inconsistent.
In ALK, two electrodes are placed in a liquid alkaline solution, usually Potassium Hydroxide (KOH). This solution helps conduct electricity necessary for the electrolysis process. The electrodes are separated by a diaphragm that keeps the gases produced from mixing and allows hydroxide ions to move between the electrodes.
Here’s what happens inside an ALK cell:
- Electrolyte: Water (H2O) breaks down into hydrogen ions (H+) and hydroxide ions (OH–).
- Cathode: Hydrogen ions gain electrons to form hydrogen gas (H2).
- Anode: Hydroxide ions lose electrons, producing oxygen gas (O2) and water (H2O).
- Overall: Water splits into oxygen and hydrogen gases.
Commercial electrolysers have many of these cells stacked together. The big challenge now is to create electrolysers that are cheaper, more energy-efficient, and can handle variable power input better.
Polymer Electrolyte Membrane (PEM)
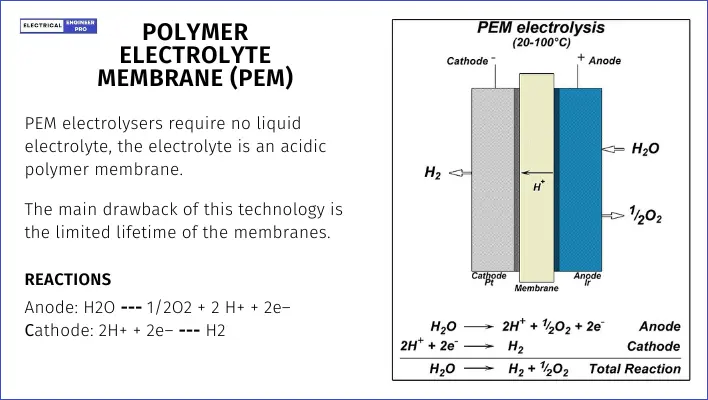
PEM electrolysis holds about 30% of the market and is popular among leading electrolyzer manufacturers. It produces higher-quality hydrogen and can be operated on and off as needed, but it’s costly and has lower production rates compared to ALK electrolysis.
PEM electrolysers are simpler because they don’t need a liquid electrolyte. Instead, they use an acidic polymer membrane.
They can potentially operate at very high pressures, making them suitable for both stationary and mobile applications. However, the main drawback is that the membranes don’t last very long.
The advantages of PEM over ALK electrolysers include a higher turn-down ratio, increased safety due to the absence of KOH electrolytes, a more compact design, and higher operating pressures.
The basic reactions at the anode and cathode in a PEM electrolyser are:
- Anode: H2O → 1/2 O2 + 2 H+ + 2e–
- Cathode: 2H+ + 2e– → H2
Despite their high cost, low capacity, poor efficiency, and short lifetimes, PEM electrolysers are not as advanced as alkaline ones. However, there’s potential for significant improvement through further materials development and cell stack design.
Also Read: Renewable Energy Systems | A comprehensive explanation
Solid Oxide Electrolyzer Cell (SOEC)
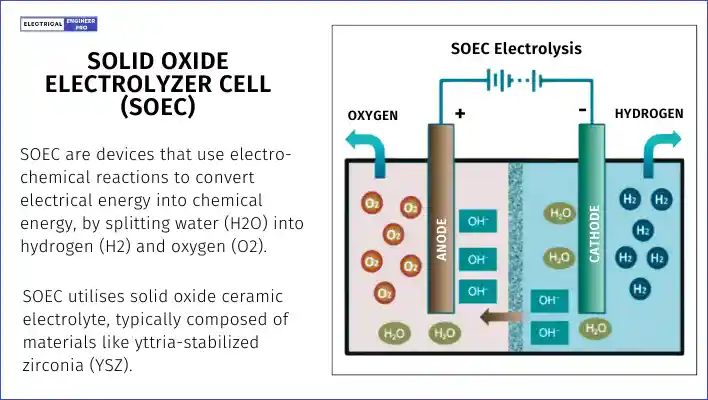
A third technology, still in the research and development stage, is the solid oxide electrolyzer cell (SOEC). This method promises high efficiency at a low cost, but it comes with challenges like a long startup time and short operational life for its components.
The SOEC is based on the solid oxide fuel cell (SOFC), typically operating at high temperatures between 700 and 1000 °C. At these temperatures, the reactions at the electrodes are more reversible, making it easier to switch from generating electricity to producing hydrogen through electrolysis.
Here’s a brief overview of the key points about SOEC:
- High efficiency and low cost
- Long startup time
- Short component lifespan
- Operates at very high temperatures
- Potential to use external heat sources to reduce electricity use
Despite these challenges, SOEC technology has the potential to become a viable option for hydrogen production with further development.
Also Read: Power of Solar and Solar Energy technologies Explained
Types of Hydrogen
Hydrogen always has to be extracted from other compounds that contain hydrogen. This means the environmental impact of hydrogen depends on how clean and eco-friendly the production method is. There are already several ways to produce hydrogen, and new methods are being developed that aim to minimize emissions.
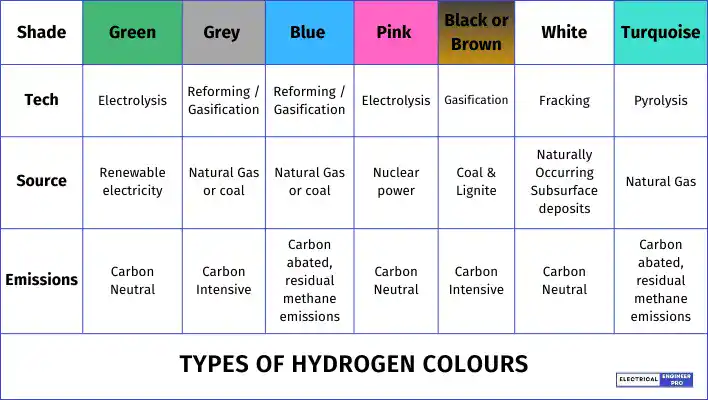
Because of this, hydrogen is categorized by different colors based on the production methods and their environmental impact:
Green Hydrogen
Green hydrogen is made by splitting water into hydrogen and oxygen using electricity from renewable sources like solar and wind power. It’s one of the cleanest ways to produce hydrogen because it doesn’t release any carbon emissions during production. This method can be scaled up easily, and as more green hydrogen projects are rolled out, the cost is expected to drop significantly, just like what happened with solar and wind energy over the past ten years.
Grey Hydrogen
Grey hydrogen is the most common type of hydrogen, but it’s known as a major climate problem because it’s made from fossil fuels. This hydrogen is produced from methane using a process called steam methane reforming (SMR) or from coal gasification. Both methods release a lot of CO2, making grey hydrogen a poor choice for achieving net-zero emissions.
Blue Hydrogen
Blue hydrogen is the second most common type and is made from natural gas or coal, similar to grey hydrogen. However, it uses carbon capture technologies like CCS (carbon capture storage) and CCU (carbon capture use) to reduce carbon emissions. The captured carbon is either stored underground, which is costly, or reused in industry, where it eventually ends up in the atmosphere.
In the long run, blue hydrogen can’t be completely carbon neutral. Even the best carbon capture technologies can only capture about 95% of emissions.
Pink Hydrogen
Pink hydrogen is made by using nuclear power to split water into hydrogen and oxygen. It’s carbon-neutral, meaning it doesn’t add to greenhouse gases. This method offers a cleaner way to produce hydrogen, using nuclear energy instead of fossil fuels or renewables. It helps solve issues linked to both traditional and renewable energy sources by providing a reliable, low-carbon alternative.
Black or Brown Hydrogen
Black or brown hydrogen is made using coal or lignite. It’s the dirtiest method, releasing 20 kilograms of CO2 for every kilogram of hydrogen produced.
White Hydrogen
White hydrogen refers to natural hydrogen deposits found underground, which can be extracted through drilling. However, these deposits are very small compared to what the world needs overall.
Turquoise Hydrogen
Turquoise hydrogen is made by heating natural gas through pyrolysis. Unlike grey or blue hydrogen, this method produces solid carbon instead of releasing it as a gas. This carbon can be used in other industries without letting it turn into CO2 later on, or it can be stored permanently to prevent emissions. However, this technology is currently expensive compared to other options, hasn’t been widely scaled up yet, and still has to address methane emissions from the natural gas used at the start.
Hydrogen Advantages
- Clean Emissions: When vehicles use hydrogen fuel cells, they only produce water as a byproduct, unlike regular cars that release harmful pollutants into the air.
- Safer Spills: If hydrogen leaks or spills, it doesn’t harm the environment or living beings like spills from fossil fuels can, which often have serious negative impacts.
- Quick Dispersion: Hydrogen quickly rises and disperses when released, which means it doesn’t linger near the ground. This reduces the risk of fire since gasoline or propane tend to stay close to the ground and pose a higher fire risk.
- Less Heat: Hydrogen flames are less hot than gasoline flames, making them less likely to cause secondary fires. This means the surrounding area doesn’t get as dangerously hot.
- Lower Explosion Risk: For hydrogen to explode, it needs a much higher concentration of oxygen compared to gasoline. This makes hydrogen a safer option in terms of explosion risks.
- Faster Fire Extinguishing: If a hydrogen fire starts, it burns up and away, and extinguishes more quickly than oil or gas fires, which tend to last longer and spread.
- Strict Safety Standards: There are strict regulations and safety standards set by organizations to ensure the safe use of hydrogen systems and fuel cells.
- Advanced Leak Detection: Even though hydrogen is colorless and odorless, modern technology can robustly detect any leaks through remote sensing, ensuring safety at hydrogen fueling stations and facilities.
Overall, hydrogen is a safer and more eco-friendly alternative to traditional fuels, supported by advanced safety technologies and stringent regulations.
Hydrogen Storage
Here are some different ways to store hydrogen:
1. Storage Tanks: These are the simplest and sometimes cheapest method. Hydrogen is typically compressed or cryogenically cooled.
The challenge with compressed hydrogen is that it needs large tanks—three times bigger than for methane and ten times bigger than for gasoline—making them more expensive due to the amount of materials needed.
Liquefying hydrogen increases its density but uses more energy—up to 30% of the hydrogen’s energy content, compared to 4-7% for compressed hydrogen.
2. Chemical Storage: This involves storing hydrogen in chemical compounds like liquid organic hydrogen carriers (LOHCs) such as methanol and toluene, or in hydrides like ammonia (NH3). Chemical storage is becoming more popular because it avoids the energy costs of liquefaction and the inefficiencies of compression.
However, each type of chemical storage has its own challenges, including the cost of converting the energy back into hydrogen and how these chemicals need to be handled safely.
3. Natural Underground Storage: Some places use salt caverns or salt domes underground to store large volumes of hydrogen at low cost. However, these options depend on local availability, which can be limited in some areas.
Green Hydrogen Uses
Green hydrogen, made by using renewable energy sources like wind or solar to split water, has many useful applications in different fields:
- Energy Storage: It can store extra renewable energy produced when demand is low, helping manage times when renewables aren’t generating.
- Transportation: It’s a clean fuel for hydrogen-powered vehicles, offering emission-free travel.
- Industry: It’s a sustainable raw material for making chemicals, refining, and producing steel, reducing the need for fossil fuels.
- Power Generation: It can generate electricity in gas turbines or fuel cells without adding to greenhouse gases.
- Heating: It can replace natural gas for heating homes and businesses, offering a cleaner option.
- Grid Stability: It helps balance the electrical grid by flexibly managing electricity generation and use.
- Off-Grid Power: It provides energy to remote places where setting up traditional power isn’t easy.
- Maritime and Aviation: It could be used in ships and planes as a cleaner alternative to conventional fuels.
These different uses show how green hydrogen helps cut down greenhouse gas emissions across various industries, making energy systems cleaner and more sustainable.
Green Hydrogen Challenges
Green hydrogen faces several significant challenges that make it hard to be widely used and integrated into our energy systems:
- High Production Costs: Right now, making green hydrogen with renewable electricity costs a lot more than producing grey hydrogen using fossil fuels. This makes it tough to compete economically, especially in sectors like fuel cell vehicles or aviation fuels, where green options are also more expensive than fossil fuels.
- Lack of Infrastructure: There’s not enough infrastructure for transporting and storing hydrogen compared to other fuels like natural gas. There are very few hydrogen pipelines globally (about 5,000 kilometers, while natural gas has over 3 million kilometers). Also, there aren’t many places to refuel hydrogen vehicles.
- Energy Losses: Making and using green hydrogen leads to significant energy losses at every step. About 30-35% of the energy used in electrolysis to create hydrogen is lost. More losses happen when hydrogen is turned into things like ammonia, moved around, and used in fuel cells. These losses mean we need even more renewable electricity to produce hydrogen, which drives up costs overall.
- Recognition and Market: Right now, there’s no separate market for green hydrogen, and it’s not clearly distinguished from grey hydrogen in global energy stats. This makes it hard for green hydrogen to compete where cutting greenhouse gases matters but isn’t rewarded financially.
- Sustainability Concerns: How green hydrogen is made depends on where the electricity comes from. Using direct renewable sources ensures green hydrogen, but if the electricity comes from fossil fuels via the grid, it undermines the environmental benefits. This variability makes it tricky to ensure hydrogen production is truly sustainable.
These challenges together stop green hydrogen from growing big, even though it could really help cut emissions in many parts of our economy. Overcoming them means investing a lot in building infrastructure, inventing new technologies, and making rules that encourage making and using green hydrogen sustainably.
Blue Hydrogen Vs Green Hydrogen
Blue Hydrogen | Green Hydrogen | |
Production Process | Produced from natural gas through steam methane reforming (SMR) or auto-thermal reforming (ATR), with carbon capture and storage (CCS). | Produced by electrolysis of water using renewable energy sources such as wind or solar power. |
Carbon Emissions | Significant emissions due to fossil fuel use. Even with Carbon Capture Storage, some emissions are generated. | Virtually zero emissions during production, as it uses renewable energy sources. |
Environmental Impact | Contributes to carbon dioxide (CO2) emissions, although less than traditional fossil fuels. | Environmentally friendly, as it does not generate greenhouse gases during production. |
Energy Source | Relies on natural gas, a fossil fuel. | Relies on renewable energy sources (wind, solar), promoting sustainability. |
Cost | Generally cheaper than green hydrogen due to mature technology and infrastructure. | Often more expensive due to the higher cost of renewable energy sources and electrolysis equipment. |
Development Stage | Well-established technology with existing infrastructure. | Emerging technology with ongoing research and development efforts. |
Applications | Widely used in industrial processes and as a feed-stock for chemicals | Primarily targeted for clean energy applications such as fuel cells and energy storage. |
Energy Efficiency | Typically lower efficiency compared to green hydrogen due to energy losses in fossil fuel extraction and conversion processes. | Higher efficiency as it directly converts renewable electricity into hydrogen. |
Scalability | Relatively easier to scale due to existing infrastructure and technology. | Faces challenges in scaling up due to the need for expanded renewable energy capacity and electrolysis facilities. |
Storage and Transport | Uses existing natural gas infrastructure for storage and distribution, which is well-established. | Requires new infrastructure for storage and distribution, especially for large-scale applications. |